Start
Part 1
ET overdosed on on Ecstasy (MDMA = methylenedioxymethamphetamine) at a rave. She become hyperthermic, and hyperventilated.
What is happening to increase her rate of oxygen consumption and her body temperature?
The increased rate of oxygen consumption and the increase in body temperature suggest that she is oxidising more metabolic fuel - we have already seen that each litre of oxygen consumed is equivalent to 20 kJ of energy expenditure - and whether this energy expenditure is associated with physical work or not, there will be an increase in heat output from the body.
What we have to consider is how it is possible for MDMA to increase the rate at which metabolic fuels are being oxidised, so leading to excessive heat production, and hence hyperthermia.
What is the main way in which the oxidation of metabolic fuels is controlled?
The aim of oxidising metabolic fuels, and hence the aim of energy yielding metabolism, is to permit the phosphorylation of ADP to ATP, so permitting the various energy requiring processes (ion transport, muscle contraction, protein synthesis and endothermic reactions) to occur. Therefore it would seem obvious that the main controlling factor over the rate at which metabolic fuels are oxidised is the need for ATP. In turn, the need to phosphorylate ADP to ATP is signalled by the availability of ADP to be phosphorylated.
So, the main factor controlling the oxidation of metabolic fuels is the utilisation of ATP in energy requiring processes.
Remember that although the total amount of (ATP = ADP) in the body is only about 5 grams, the total amount of ATP formed from ADP, and the total amount of ATP utilised each day is about equal to body weight, some 70 kg.
How can we measure the rate at which metabolic fuels are being oxidised?
We have seen in the jogging exercise that total energy expenditure can be measured by measuring oxygen consumption - regardless of the mixture of fuels being oxidised, each litre of oxygen consumed is equivalent to expenditure of 20 kJ. Therefore we can determine the rate at which fuels are being oxidised by measuring oxygen consumption.
We can measure oxygen consumption in the whole body, in isolated cells and tissues, and indeed in isolated subcellular compartments (the mitochondrion is the main subcellular organelle that consumes oxygen). This means that we can investigate energy metabolism and he oxidation of fuels not only in the whole body but also in isolated cells and tissues, and isolated mitochondria.
If we want to consider the oxidation of metabolic fuels linked to formation of ATP from ADP and inorganic phosphate, we need to consider the steps involved.
What are the chemical processes of oxidation and reduction?
Does oxidation necessarily involve oxygen?
Chemically, oxidation is the process of removing electrons from a molecule - either electrons alone or, more commonly with organic compounds, removal of electrons and hydrogen ions (protons), so that oxidation of organic compounds is the removal of hydrogen. The addition of oxygen (from oxygen itself or from water) is also oxidation.
Reduction is the reverse - the addition of electrons, either alone or, more commonly with organic compounds, addition of electrons and hydrogen ions (protons), so that reduction of organic compounds is the addition of hydrogen. The removal of oxygen (commonly as water) is also reduction.
The addition or removal of electrons, alone or together with hydrogen ions, does not involve oxygen.
How do metabolic fuels, which are organic compounds undergo oxidation if oxygen is not directly involved?
The various oxidative reactions in metabolism are all linked to the reduction of coenzymes, by transferring electrons and hydrogen ions from the substrate to the coenzyme. There are two groups of coenzymes involved:
Nicotinamide adenine dinucleotide (NAD) and nicotinamide adenine dinucleotide (NADP).
In these two compounds the nicotinamide ring has a positive change on the nitrogen in the oxidised form. In reduction, one electron neutralises this positive charge, and its associated proton remains associated with the reduced coenzyme; the other electron with its associated proton is incorporated into the ring.
Note that there is one hydrogen at position 4 of the ring in the oxidised coenzymes, but by convention this is not shown.
There is only a small amount of NAD and NADP in cells - like other coenzymes, there is a very high rate of turnover of a small amount of coenzyme. The reduced coenzymes formed in the oxidation of substrates are rapidly re-oxidised, either in reactions where a substrate is reduced (this is the main role of NADPH), or in the mitochondria, linked to the phosphorylation of ADP to ATP.
The flavin coenzymes
The flavin coenzymes are derived from the vitamin riboflavin (vitamin B2). In some cases riboflavin itself is covalently bound at the active site of the enzyme; such proteins are generally called flavoproteins. In other cases either riboflavin phosphate (also known as flavin mononucleoride) or flavin adenine dinucleotide is tightly (but not covalently) bound at the active site of the enzyme. The reduced flavins are rapidly reoxidised in the mitochondria, linked to the phosphorylation of ADP to ATP.
Note that while the oxidation and reduction of NAD and NADP shown above is always a two electron process (transferring two electrons and two protons), the oxidation and reduction of flavin coenzymes can occur either as a two electron reaction (transferring two electrons and two protons at the same time) or as two separate single electron steps (transferring one electron and one proton at a time), with intermediate formation of the flavin semiquinone radical.
How is oxygen consumed in the oxidation of metabolic fuels if the substrates are oxidised by reducing NAD and flavin coenzymes?
The reduced coenzymes are reoxidised in the mitochondrial electron transport chain, a series of coenzymes or electron carriers in the crista membrane of the mitochondrion. Each carrier is reduced by the one above it in the chain, then re-oxidised by reducing the next one along. The final carrier is re-oxidised by reducing oxygen to water.
There are three sites in this sequence of electron carriers that are associated with the phosphorylation of ADP to ATP.
Note that electrons from substrates that reduce flavins enter the chain part-way down, after the first site at which ATP formation occurs. This means that there are three sites available for ATP formation in the oxidation of substrates that reduce NAD, but only two in the oxidation of substrates that reduce flavins.
How can you measure the rate of metabolism in isolated mitochondria?
The final step in the electron transport chain is the reduction of oxygen to water, so you can measure the rate of metabolism by measuring the rate of consumption of oxygen. This is done by incubating mitochondria in a sealed vessel containing an oxygen electrode - an electrochemical way of measuring oxygen. Initially the buffer is more or less completely saturated with oxygen, and as oxygen is reduced to water, so the saturation of the buffer with oxygen falls. The results are shown as a graph of the percentage saturation with oxygen against time.
What else must you add to the mitochondria to see oxygen consumption?
Obviously, you need to add a substrate - conventionally either of two substrates is used:
- malate, which is oxidised to oxaloacetate by reducing NAD
- succinate, which is oxidised to fumarate by reducing a flavin
Perhaps less obviously, you also have to add ADP. There are three sites in the electron transport chain from NADH (and two in the chain from FADH) at which ATP is formed. This linkage between electron transport and phosphorylation of ADP is obligatory, and if there is no ADP available to be phosphorylated, electron transport comes to a halt. This means that oxygen is not consumed, and substrates are not oxidised.
The graph on the right shows the effect on oxygen consumption of adding increasing amounts of ADP - the more ADP is added, the more ATP can be formed, and the more oxygen is consumed (and hence also the more substrate is oxidised). The oxidation of substrates and consumption of oxygen is limited by the availability of ADP.
The graph also shows that there is only a very small consumption of oxygen after the mitochondria have been added, because there is only a very small amount of ADP available in the mitochondria before it has been added.
Part 2
The graph shows the oxygen electrode traces for two separate experiments, superimposed on each other. In both experiments there was the same amount of ADP added; one experiment used 2500 nmol of malate as the substrate and the other used 2500 nmol of succinate.
Can you explain why the incubation with succinate used more oxygen to phosphorylate the same amount of ADP?
Succinate reduces a flavin, whereas malate reduces NAD.
There are three sites associated with ATP formation when NADH is re-oxidised, but only two when reduced flavin is re-oxidised.
The consumption of oxygen is controlled by the amount of ADP present.
If malate is being metabolised, there are three sites at which ADP is phosphorylated to ATP for each NADH that is oxidised, and one atom of oxygen is consumed to form water.
If succinate is being metabolised, there are only two sites at which ADP is phosphorylated to ATP for each reduced flavin that is oxidised, and one atom of oxygen is consumed to form water.
Therefore for the same amount of ADP to be phosphorylated to ATP when succinate is the substrate, more succinate will be used, meaning that more oxygen will be used.
This suggests that the ratio of ATP formed : oxygen consumed (the P:O ratio) should be 3 when malate is the substrate, and 2 when succinate is the substrate. However, no matter how carefully you prepare the mitochondria and how carefully you perform the experiments, what you observe is that the P:O ratio for malate is ~2.5 and for succinate is ~ 1.5.
Can you explain why the P:O ratio is not what you would expect, and why it is not a whole number?
We now have to look at the electron transport chain in a little more detail.
Click here to see the structures of the other carriers of the electron transport chain.
Looking at complex 1 in the red box:
NADH is oxidised by reducing an oxidised flavin to the fully reduced flavin.
The reduced flavin is re-oxidised in two steps (via the flavin semiquinone) by reducing the iron atoms in two molecules of a protein that contains iron bound to sulphur (a non-haem iron protein, so called because unlike the cytochromes, it contains bound iron that is not in a haem ring).
The reduced iron is re-oxidised by reducing ubiquinone to ubiquinol.
This raises an interesting problem. NAD, flavin and ubiquinone are all reduced by transferring both hydrogen ions and electrons. By contrast, iron is reduced by transferring electrons alone.
What happens to the protons when iron is reduced by the reduced flavin?
Where do the protons come from when ubiquinone is reduced by the iron sulphur protein?
The carriers are all arranged in the christa membrane of the mitochondrion. Some are at the inner surface, facing the matrix of the mitochondrion, some are at the outer surface, facing the crista space, and some span the membrane.
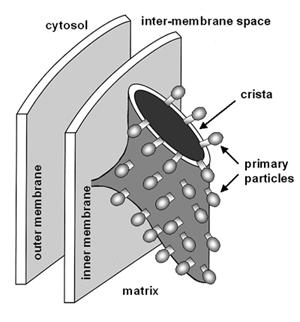
Looking at complex 1 in more detail we can see that NADH is oxidised by the flavoprotein at the inner surface of the membrane, and the reduced flavin is re-oxidised by the non-haem iron protein at the outer surface of the membrane. The protons that were carried by the flavin and are not required to reduce the iron are ejected into the crista space.
The non-haem iron protein is re-oxidised by reducing ubiquinone at the inner surface of the membrane - and the protons that are required to reduce ubiquinone are acquired from water in the mitochondrial matrix.
This means that as electrons pass from NADH to ubiquinone, protons are pumped into the crista space.
There is similar pumping of protons across the membrane into the crista space in complexes III and IV as well, and overall the oxidation of 1 mol of NADH leads to the transfer of 11 protons from the matrix into the crista space, creating a pH gradient across the membrane.
Fewer protons are pumped across the membrane when reduced flavin is oxidised because the pumping site in complex I is not involved in the oxidation of reduced flavins, which reduce ubiquinone directly.
How does this pH gradient drive the phosphorylation of ADP to ATP?
The formation of ATP by condensation between ADP and inorganic phosphate is a highly endothermic reaction. The ATP synthetase is located in the primary particles on the inner face of the crista membrane; isolated primary particles catalyse hydrolysis of ATP to ADP and phosphate, and you will sometimes see ATP synthetase referred to as an ATPase.
ATP synthetase is a multi-subunit enzyme with three equivalent catalytic sites.
At any given time, each site is at a different stage in the reaction:
- One site is binding ADP and phosphate
- One site is catalysing the condensation of ADP and phosphate, and expelling and water
- One site is expelling ATP, ready to accept ADP and phosphate
As protons enter through the stalk of the primary particle that spans the crista membrane, so they cause a rotation of the central part of the ATP synthetase, forcing each site in turn to proceed to the next step in the reaction.
The ATP synthetase acts like a molecular motor, driven by the flow of protons down their concentration gradient in much the same way as a water mill is driven by the flow of water in the mill stream. The rate at which it turn, and hence the amount of ATP formed will depend on the rate at which protons are flowing through the central core, not the number of protons that have crossed the membrane.
Can you explain how it is that electron transport and substrate oxidation are controlled by the availability of ADP?
If there is no ADP available to bind to the empty site, then rotation of the central part of the ATP synthase is not possible, and protons cannot cross the stalk of the primary particle. This leads to an accumulation of protons in the crista space, which, in turn, inhibits any further expulsion of protons by the electron transport chain, so that electron transport (and therefore substrate oxidation) ceases.
Can you explain why the P:O ratios are ~2.5 for oxidation of malate and ~1.5 for the oxidation of succinate?
It is difficult to account for these ratios if we think in simple chemical terms. However, as we saw above, The ATP synthase acts like a molecular motor, driven by the flow of protons down their concentration gradient in much the same way as a water mill is driven by the flow of water in the mill stream. The rate at which it turn, and hence the amount of ATP formed will depend on the rate at which protons are flowing through the central core, not the number of protons that have crossed the membrane.
What would you expect to see if the mitochondrial crista membrane could be made permeable to protons?
If something could be provided to form a proton pore through the membrane then most of the protons would re-enter the matrix through this pore, and not through the ATP synthase.
This would mean that electron transport could proceed at a very fast rate, not limited by the availability of ADP, until all the oxygen or substrate was depleted - normally the factor that controls the amount of oxygen that is used is the amount of ADP available, and when ADP is depleted oxygen consumption ceases, even if there is still oxygen available.
Compounds that render the mitochondrial membrane permeable to protons are called uncouplers, because the break the usual tight and obligatory coupling between electron transport and ATP synthesis, permitting rapid and uncontrolled electron transport and oxygen utilisation.
The graph shows the oxygen electrode traces for two separate experiments, superimposed in each other. In both experiments there was the same amount of ADP added, with malate as the substrate. In one experiment an uncoupler was added, the other was the control incubation.
What type of chemical compound might make the membrane permeable to protons and so act as an uncoupler?
A weak acid that is lipid soluble when protonated could transport protons across the membrane - the first such compound to be found to act as an uncoupler was dinitrophenol.
Dinitrophenol will cross the outer membrane freely, as do many compounds. The intermembrane space is contiguous with the crista space. In the crista space dinitrophenol will be protonated, because of the relatively high concentration of protons that have been pumped out by the electron transport chain. protonated dinitrophenol is lipid soluble and will therefore dissolve in the membrane lipid, down a concentration gradient.
In the matrix the dinitrophenol will be deprotonated, because of the relative excess of hydroxyl ions. This means that there will continue to be an inwards concentration gradient for protonated dinitrophenol, and protons will be transported inwards until the proton gradient has been abolished.
There are uncoupling proteins in muscle and brown adipose tissue that act in the same way as dinitrophenol, in that they transport protons back across the crista membrane, bypassing the ATP synthase.
What do you think is the physiological function of these uncoupling proteins?
There are uncoupling proteins in muscle and brown adipose tissue that act in the same way as dinitrophenol, in that they transport protons back across the crista membrane, bypassing the ATP synthase.
If the proteins uncouple electron transport from the phosphorylation of ADP (to a limited extent) when the are activated, this will allow some oxidation of metabolic fuels to occur without forming ATP - resulting in increased heat production.
The first such protein to be identified was in brown adipose tissue, and was called thermogenin, since it permits heat production (thermogenesis) without shivering (which is muscle contraction to no useful purpose other than to generate heat). This non-shivering thermogenesis in brown adipose tissue is known to be important in infants to maintain body temperature. It is also important in hibernating animals, whose rate of metabolism and body temperature fall while they are hibernating - shortly before the wake up from hibernation their brown adipose tissue thermogenin is activated, increasing their metabolic rate and raising their body temperature.
It is not known to what extent brown adipose tissue is normally active in adults, but a number of studies using heat-sensitive cameras have shown that stimulation of thermogenin leads to increased temperature of regions of the body where there are deposits of brown adipose tissue.
Brown adipose tissue is so-called because of its brown colour; the main adipose tissue reserves of the body are white adipose tissue, which acts to store triacylglycerol, and is white or yellow in colour. The brown colour is due to the cytochromes in the large number of mitochondria in brown adipose tissue.
After the discovery of thermogenin in brown adipose tissue, uncoupling proteins were also discovered in skeletal muscle. Again presumably they are involved in the maintenance of body temperature.
ET overdosed on on Ecstasy (MDMA = methylenedioxymethamphetamine) at a rave. She become hyperthermic, and hyperventilated.
From what you have learnt in this exercise, can you suggest how MDMA acts to raise body temperature and increase oxygen consumption?
From its structure, MDMA is obviously not a weak acid like dinitrophenol, so it cannot act as an uncoupler directly. Its most likely mechanism of action is that it activates thermogenin in brown adipose tissue and / or uncoupling proteins in skeletal muscle.
Why do you think that dinitrophenol was used at one time as a slimming agent?
If you uncouple electron transport from phosphorylation of ADP to a limited extent you will metabolise metabolic fuels at a greater rate than normal, since the rate of oxidation is now less controlled by the availability of ADP to be phosphorylated. This means there will be an increase in metabolic rate and triacylglycerol stores in adipose tissue will be metabolised to a greater extent than normal. You could call this an "armchair" or non-exercise form of exercise, since it acts in a similar way to exercise to increase metabolic rate, and hence increase consumption of metabolic fuel.
Dinitrophenol is no longer used as a slimming drug - it is toxic.
What would you observe in someone who had taken a modest dose of dinitrophenol?
The same as in ET, who overdosed on MDMA - an increase in body temperature and hyperventilation to breathe in more oxygen to permit the oxidation of more metabolic fuel.
Key points from this exercise:
- The aim of oxidising metabolic fuels, and hence the aim of energy yielding metabolism, is to permit the phosphorylation of ADP to ATP, so permitting the various energy requiring processes (ion transport, muscle contraction, protein synthesis and endothermic reactions) to occur.
- The main factor controlling the oxidation of metabolic fuels is the availability of ADP, reflecting the utilisation of ATP in energy requiring processes.
- Chemically, oxidation is the process of removing electrons from a molecule - either electrons alone or, more commonly with organic compounds, removal of electrons and hydrogen ions (protons), so that oxidation of organic compounds is the removal of hydrogen.
- Reduction is the reverse - the addition of electrons, either alone or, more commonly with organic compounds, addition of electrons and hydrogen ions (protons), so that reduction of organic compounds is the addition of hydrogen.
- Metabolic fuels undergo oxidation by removal of hydrogen (protons and electrons) by transfer to an intermediate hydrogen carrier - either the nicotinamide nucleotides NAD and NADP or a flavin coenzyme.
- Cells contain very small amounts of these coenzymes, which are rapidly re-oxidised in the mitochondrial electron transport chain.
- Oxygen is not directly involved in the oxidation of metabolic fuels, but the final reaction of the electron transport chain is the reduction of oxygen to water.
- Measurement of oxygen consumption provides a way of following mitochondrial metabolism.
- Mitochondria do not consume a significant amount of oxygen, even if substrate is added, unless ADP is also added. The process of substrate oxidation and oxygen consumption is obligatorily linked to the phosphorylation of ADP to ATP.
- Malate reduces NAD, and there are three sites associated with phosphorylation of ADP to ATP when NADH is re-oxidised in the mitochondrial electron transport chain. Succinate reduces a flavin, and there are only two sites associated with phosphorylation of ADP to ATP when reduced flavin is re-oxidised in the mitochondrial electron transport chain. This means that for the same amount of ADP phosphorylated to ATP, more substrate will be oxidised, and more oxygen will be consumed, when succinate is the substrate than when malate is the substrate.
- The electron transport chain consists of a series of compounds, each of which is reduced by the carrier preceding it, and is reoxidised by reducing the next carrier in the chain. The final carrier is reoxidised by reducing oxygen to water.
- The ratio of ADP phosphorylated : oxygen consumed (the P:O ratio) is not an integer; when malate is the substrate the ratio is ~2.5, and when succinate is the substrata the ratio is ~1.5.
- Eleven protons are pumped across the crista membrane into the crista space as the carriers of the electron transport chain from NADH undergo reduction and re-oxidation.
- These protons re-enter the mitochondrial matrix through a membrane spanning region of the ATP synthase. As they do so, they cause the core of the enzyme to rotate, forcing the active sites of the enzyme to catalyse the next stage of the reaction of ATP synthesis. The enzyme has three active sites, and at any time, one is binding ADP and phosphate, one is catalysing the condensation to form ATP and the third is expelling ATP.
- Weak acids render the crista membrane permeable to electrons, so uncoupling electron transport from oxidative phosphorylation and permitting uncontrolled oxidation of substrate until all the available oxygen has been consumed.
- Uncoupling proteins in brown adipose tissue and muscle are important in maintenance of body temperature - the process of non-shivering thermogenesis.