Start
AP is a 16 year old boy. As an infant he was liable to convulsions if he was not fed at regular intervals. He has a greatly enlarged liver, which is readily palpable. For the last year he has suffered increasingly from gout, with deposition of crystals of uric acid in joints.
The following results were obtained on blood samples taken after an overnight fast:
glucose (mmol /L) |
insulin (mU /mL) |
non-esterified fatty acids (mmol /L) |
|
AP | 2.2 |
2.0 |
2.08 |
10 age-matched control subjects | 4.0 ± 0.16 |
9 ± 0.7 |
0.71 ± 0.08 |
What conclusions can you draw from these results?
He has very low plasma glucose in the fasting state. Indeed, this is close to the level at which we might expect to see disturbance of consciousness or convulsions.
His plasma insulin is very low, presumably as a result of the low circulating concentration of glucose. It is unlikely that his problem is failure to secrete insulin (i.e. diabetes mellitus), since in this case we would expect to see a high plasma concentration of glucose with low insulin.
His plasma free fatty acids are very high in the fasting state. This would be expected from the low plasma glucose, since fatty acids will be mobilised from adipose tissue to provide an alternative fuel. We are not given any data, but we would expect his plasma ketone bodies (acetoacetate and hydroxybutyrate) to be high as well.
Although neither the enlarged liver nor the hypoglycaemia with low plasma insulin is typical of diabetes mellitus, in order to be sure he was given an oral dose of 1 g of glucose /kg body weight, and his plasma glucose was measured over 4 hours afterwards - a standard oral glucose tolerance test.
What conclusions can you draw from the results of the glucose tolerance test?
The response to a 50g test dose of glucose clearly eliminates the possibility of diabetes mellitus; in diabetes we would expect to see a very much greater than normal rise in plasma glucose, to considerably above 10 mmol/L, remaining high for much longer. Certainly we would not expect to see the sharp fall in plasma glucose 3½ - 4 h after the test dose. The patient is able to maintain plasma glucose near the normal range after the test dose, so there is no impairment of his ability to take up glucose into tissues, either for oxidation as a metabolic fuel or for glycogen synthesis.
50g of glucose yields 800 kJ; the average energy expenditure of a 16y old boy is 9 - 9.5 MJ/day; allowing for his initially high plasma free fatty acids, it is to be expected that by 3½ - 4 hours after 50g of glucose he would again be in a fasting state.
What is abnormal is that his plasma glucose again falls to a very low level. You would normally expect plasma glucose to be maintained within the normal range by utilisation of the liver glycogen that has been synthesised in response to the glucose load.
A liver biopsy was taken from AP after an overnight fast, and from a control subject of the same age who was being investigated for an unrelated condition. The dark granules in the electron micrographs below are glycogen granules.
What conclusions can you draw from this liver biopsy?
The liver histology shows that the gross enlargement of AP's liver was probably due to accumulation of abnormally large amounts of glycogen.
After an overnight fast most of the liver glycogen will normally have been used to maintain plasma glucose the micrograph on the right, from the normal subject, shows few dark-staining glycogen granules. By contrast the AP’s biopsy sample shows a very large amount of glycogen even after an overnight fast, when he was very hypoglycaemic.
It is possible that his problem is due to a failure to mobilise liver glycogen to maintain plasma glucose in the fasting state; there is obviously no defect in his ability to synthesise glycogen. The results are not shown, but his muscle glycogen was normal.
AP's plasma concentration of lactate after an overnight fast was abnormally high, so lactate was measured in the blood samples taken during his glucose tolerance test. The results are shown in the graph on the right.
Why do you think his plasma lactate was high after an overnight fast?
AP's very high plasma lactate in the fasting state suggests that he is able to mobilise muscle glycogen to glucose 1-phosphate; in even moderate exercise a considerable amount of muscle glycogen is metabolised to lactate, which is released into the bloodstream. This is more marked in the fasting state. The liver takes up this lactate for gluconeogenesis, normally releasing glucose into the bloodstream.
Like the glucose alanine cycle (see the exercise on Alanine released from muscle in fasting), this anaerobic glycolysis of muscle glycogen provides an indirect way in which muscle glycogen can contribute to maintenance of the plasma glucose concentration in fasting.
What conclusions can you draw from these results?
In response to oral glucose, AP's plasma lactate falls slowly, as it is cleared by the liver. Since he has a normal plasma concentration of glucose during the first 3 hours after the glucose load, there is little release of lactate from the muscle after the glucose load. Insulin stimulates the uptake of glucose into muscle, where it is used for glycogen synthesis.
The glucose formed by gluconeogenesis form lactate will also be used for glycogen synthesis in the liver - for as long as his plasma concentration of glucose is relatively high, the liver will not release glucose formed by gluconeogenesis, but, stimulated by insulin, will synthesise glycogen.
What we still cannot explain is why he is unable to mobilise this glycogen later to maintain normoglycaemia, so that his plasma glucose falls precipitously between 3.5 and 4 hours after the glucose dose.
Normally in the fasting state, glucagon stimulates the phosphorolysis of glycogen in liver, leading to the sequence of reactions shown below:
Glycogen phosphorylase is activated by phosphorylation of the enzyme, catalysed by phosphorylase kinase, which is stimulated by cyclic AMP released in response to binding of glucagon to cell surface receptors.
Therefore, AP was given an injection of 1 mg of glucagon after an overnight fast, and his plasma glucose was measured over 2 hours after the injection. The results are shown in the graph on the right.
What conclusions can you draw from these results?
AP is unable to respond to glucagon in a normal way; there is no increase at all in his plasma glucose, which continues to fall, despite the injection of glucagon.
Four possible defects may account for this:
- His glucagon receptors may be defective.
- Lack of phosphorylase kinase, thus preventing the activation of glycogen phosphorylase in response to glucagon action.
- Lack of glycogen phosphorylase, thus preventing the mobilisation of glycogen to glucose 1-phosphate in liver and muscle. However, we can eliminate this possibility because he does seem to be able to mobilise muscle glycogen in the fasting state, producing a relatively large amount of lactate.
- Lack of glucose 6-phosphatase, thus preventing the hydrolysis of glucose 6-phosphate to free glucose in the liver.
Like glucagon, adrenaline acts to stimulate glycogenolysis in the liver, again acting via cyclic AMP and phosphorylase kinase. Adrenaline also activates hormone-sensitive lipase in adipose tissue, leading to the release of non-esterified fatty acids.
AP was given an injection of 15 µg of adrenaline /kg body weight, and both glucose and non-esterified fatty acids were measured over 3 hours after the injection. The results are shown in the graphs below:
What conclusions can you draw from these results?
The graph on the left shows that he is unable to mobilise glycogen and release free glucose from the liver in response to adrenaline.
Four possible defects may account for this:
- His liver adrenaline receptors may be defective. However, we know from the graph on the right that his adipose tissue adrenaline receptors are normally responsive, since there is a considerable increase in plasma non-esterified fatty acids in response to adrenaline. It is highly unlikely that both adrenaline and glucagon receptors are abnormal in the liver, but normal in adipose tissue.
- Lack of phosphorylase kinase, thus preventing the activation of glycogen phosphorylase in response to adrenaline action.
- Lack of glycogen phosphorylase, thus preventing the mobilisation of glycogen to glucose 1-phosphate in liver and muscle, but this has already been eliminated as a possibility.
- Lack of glucose 6-phosphatase, thus preventing the hydrolysis of glucose 6-phosphate in the liver to release free glucose.
We are left with two possible explanations for his excessive storage of glycogen in the liver:
- Lack of phosphorylase kinase
- Lack of glucose 6-phosphatase
Both galactose and fructose can be phosphorylated by hexokinase, and fructose 6-phosphate and galactose 6-phosphate can be isomerised to glucose 6-phosphate. Therefore AP was given an intravenous infusion of 1 g of galactose /kg body weight, and, on another occasion, 0.5 g fructose /kg body weight. In each case the infusion lasted for 5 minutes, shown by the yellow bar, and plasma glucose was measured over 30 minutes from the start of the infusion. The results are shown below:
What conclusions can you draw from these results?
Neither galactose nor fructose leads to an increase in plasma glucose. A defect of glycogen phosphorylase or phosphorylase kinase would not affect the formation of glucose from fructose or galactose; neither would insensitivity of the liver to adrenaline and glucagon.
These graphs confirm that the most likely defect is in glucose 6-phosphatase.
AP suffers for glycogen storage disease type I (von Gierke's disease) - genetic lack of glucose 6-phosphatase in liver, leading to profound hypoglycaemia on fasting, and gross enlargement of the liver as a result of accumulation of glycogen that cannot be mobilised.
Until such time as gene therapy is possible, how might you treat him?
Obviously he needs to avoid conditions under which he will synthesise large amounts of glycogen that he cannot use. This means that he will require frequent small meals, so as to avoid high glucose concentrations that will lead to glycogen synthesis. His fasting hypoglycaemia can probably best be treated by slow infusion of glucose or small glucose polymers, through a naso-gastric tube through the night - the same technique as is used to enhance nutritional status in children with cystic fibrosis.
We now have to consider why AP suffers from gout at such a young age.
A number of conditions that lead to persistent high blood concentrations of lactate are associated with gout, which is due to high blood levels of uric acid, which has a low solubility and crystallises in joints, causing severe pain and inflammation.
The graph below shows the effect of an infusion of sodium lactate (a total of 1 L of 0.4 mol /L sodium lactate was infused over 2 hours) on renal clearance of uric acid in a healthy control subject.
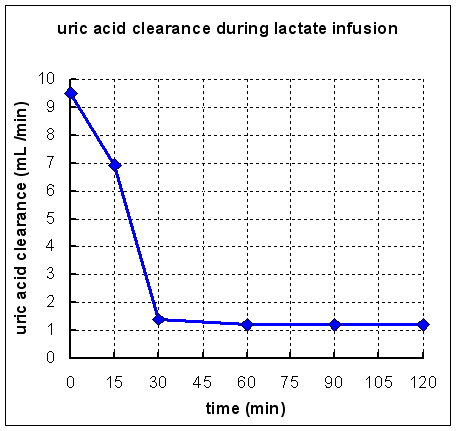
What conclusions can you draw from these results?
Obviously lactate inhibits the renal clearance, and hence the excretion, of uric acid.
Uric acid excretion is unusual, in that it is filtered in the glomerulus, more or less completely reabsorbed in the proximal renal tubule, and actively secreted in the distal renal tubule. It is likely that lactate inhibits the active secretion of uric acid in the distal renal tubule.
Key points from this exercise:
- In the fasting state muscle glycogen can be an indirect source of blood glucose as a result of anaerobic glycolysis in muscle, with the release of lactate into the bloodstream, and gluconeogenesis from this lactate in the liver.
- In healthy people, injection of glucagon results in mobilisation of glucose from liver glycogen and elevation of blood glucose concentration.
- Failure of glucagon injection to raise blood glucose may be due to a number of possible defects:
- defective glucagon receptors
- lack of glycogen phosphorylase
- lack of phosphorylase kinase
- lack of glucose 6-phosphatase
- In healthy people, injection of adrenaline results in mobilisation of glucose from liver glycogen and elevation of blood glucose concentration, as well as increased lipase activity in adipose tissue, leading to release of non-esterified fatty acids into the bloodstream.
- Failure of adrenaline injection to raise blood glucose may be due to a number of possible defects:
- defective adrenaline receptors
- lack of glycogen phosphorylase
- lack of phosphorylase kinase
- lack of glucose 6-phosphatase
- Both galactose and fructose are phosphorylated by hexokinase, and galactose 6-phosphate and fructose 6-phosphate are isomerised to glucose 6-phosphate, which can normally be hydrolysed to release free glucose into the bloodstream.
- Lack of glucose 6-phosphatase in liver leads to accumulation of glycogen that cannot be utilised, and hence to profound fasting hypoglycaemia, as well as persistent lactic acidosis.
- Persistent lactic acidosis can lead to the development of gout, because lactate inhibits the renal clearance of uric acid.
- Type I glycogen storage disease, due to lack of glucose 6-phosphatase, can be treated by feeding small frequent meals, so as to maintain a more or less constant blood concentration of glucose, without it rising so high that glycogen synthesis is activated.